August 31, 2023
“I'm sorry, but I just don't understand anything in biology unless I know what it looks like.”
Don C. Wiley
From the organism to the organ, the cell to the organelle, the molecule to the atom, biologists have descended into the living matter not only describing it but seeing and photographing it. Whether to convince by their explanatory power or compel by their beauty, images of biological phenomena have transformed how we approach the field. The story of how humanity created devices to study living organisms is the story of how we came to understand ourselves, pushed by the desire to see in more detail. The evolution of the microscope is a prime example of technical advances in lockstep with biological discoveries.
The Early Days
While rudimentary single-lens microscopes were used in the antique world, real progress in performance was only made in the 16th and 17th centuries with the invention of the light microscope. The two main ways microscopes were improved at the time were by (1) adding a second set of lenses to concentrate the light into the specimen (thus making compound microscopes), and (2) improving the quality of the lenses to remove optical flaws. While the Dutch spectacle maker Zacharias Janssen made one of the earliest compound microscopes, it was Antonie van Leeuwenhoek (who we referenced in our Notes on Fermentation) who was able to magnify objects by up to 200 times when the earlier microscopes were limited to about 30 times. He likely was the first to bring this new technological prowess to the attention of natural scientists interested in studying living things — people we now call “biologists”). With no scientific training, this draper produced hundreds of high-quality single-lens microscopes and communicated his observations of single-cell organisms through letters to the Royal Society. Van Leeuwenhoek’s scientific interest was borne out of seeing its subject, and the power to evoke its natural beauty has continued to inspire generations of scientists. A contemporary of van Leeuwenhoek, Robert Hooke, published Micrographia in 1665, making observations and describing some strange “pores” when looking at thin slices of cork under the microscope: "all perforated and porous, much like a Honey-comb, ... these pores, or cells, ... were indeed the first microscopical pores I ever saw."

The Birth of Cell Biology
Unknowingly, Hooke’s first mention of the word “cell” kicked off the development of a new scientific field: cell biology. Over the next two hundred years, the improvement in lens manufacturing and the addition of mirrors helped solve early problems such as color separation (chromatic aberration) and image blurring (spherical aberration). These improved microscopes played a central role in the development of cell theory by two German scientists, Mathias Schleiden and Theodor Schwann, who proposed that cells were the building blocks for plant and animal life. By this time, the compound microscope was standard scientific equipment in labs and an indispensable pedagogical tool. These early microscopes allowed delving into organisms and describing their different cell types based on their morphology and position within a tissue. The next wave of inventions would enable biologists to dive deeper into the mechanisms of living organisms and enter inside cells.
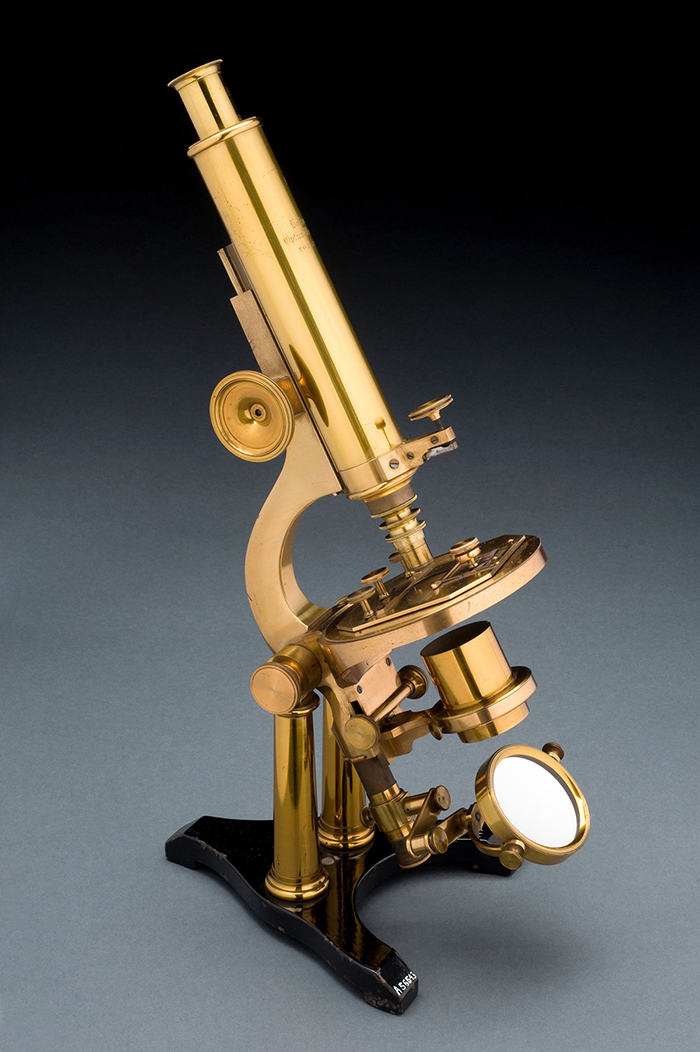
The Leaps Forward
A real challenge in observing biological samples is how to direct light to distinguish the specimen from its aqueous surroundings, given their very close refractive indexes. The techniques in sample illumination are fairly recent and at least two revolutionized how we can now see biological samples:
Phase-contrast microscopy
This technique permits the gain of contrast in a translucent specimen, such as mammalian cells, without staining. The optical methodology was introduced by Zernicke in 1932, for which he was awarded the Nobel Prize in 1953. This technique exploits the fact that light slows slightly when passing through biological specimens and allowed for much finer description of cell morphology.


Fluorescence microscopy
No other advancement since the invention of the microscope had such a dramatic effect on the study of biology as the invention of fluorescence microscopy. While antibody-targeted immunofluorescence to increase contrast was developed in the late 19th century, the field was transformed by the discovery of the Green Fluorescent Protein (GFP), isolated from a jellyfish in 1962. After working out how the protein emitted light upon excitation by a laser, the gene coding for the protein was sequenced and expressed outside the jellyfish. In 1994, the gene was then incorporated into the promoter for a gene that encoded β-tubulin and showed that it could serve as a marker for expression levels. Osamu Shimomura, Martin Chalfie, and Roger Tsien were recognized in 2008 with the Nobel Prize in chemistry for their work on GFP. Later on, the protein was mutated to manufacture blue, cyan, yellow, and many more derivatives, all the way to the technical prowess of staining hundreds of individual neurons with different colors, creating a “Brainbow” mouse. Fluorescence microscopy launched the production of extraordinary images, powerful both by their beauty and explanatory abilities.
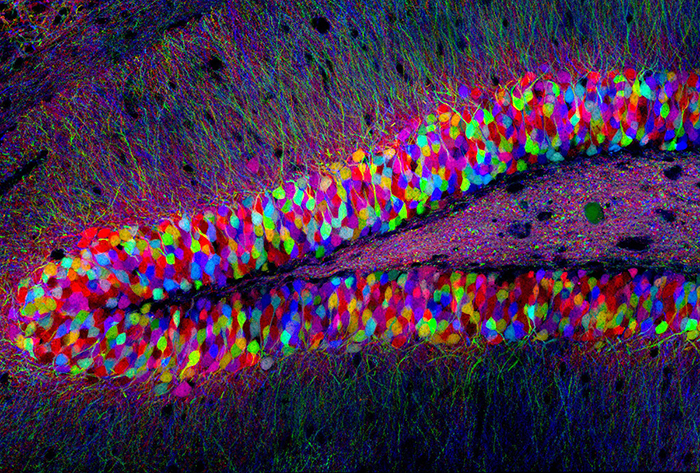
The Impossible, Overcome
The theoretical and technical limitations of the light microscope were fully described as early as the 19th century by Ernst Abbe. While the human eye can distinguish objects of about 100um (1/10 of a millimeter or the size of a human hair), it was posited that the theoretical resolution of a light microscope was limited to around 200nm (or the size of a mitochondrion), due to diffraction properties. It is partly to overcome these limitations that electron microscopes were developed, enabling a far greater resolution to around 1Å (1/1000 nm), close to the size of the atom. While electron microscopy has produced some key discoveries, one of its unavoidable flaws is that there is no way to observe live tissues and cells. In the late 1990s, through remarkable inventiveness, Stefan Hell managed to circumvent the Abbe diffraction limit, thought unbreakable for over a hundred years, leading the way to super-resolution microscopy and a well-deserved Nobel Prize in 2014. In Hell’s path, a series of new techniques both computational and physical allowed biologists to follow single molecules in live cells.


Earthrise, the picture of the Earth taken from the moon’s surface by the Apollo 8 crew, had an emotional impact on people, encouraging them to engage with environmental issues. Similarly, the ability to see living systems with ever more clarity and resolution hastransformed our understanding of biology, and continues to push our research guided by a sense of awe driven by the power of the image.
– Jonathan Friedlander, PhD & Geoffrey W. Smith
Engineering Biology
Jacob Oppenheim, PhD, an Entrepreneur-In-Residence at Digitalis Ventures, writes Engineering Biology at Digitalis Press:
This month, Jacob discussed how the past three decades of scientific advances in biology have given us a newfound power to engineer biological systems and map their underlying primitives universally, rather than relying on serendipity and reasoning by analogy. These changes make it possible for us to digitize and standardize biopharmaceutical research + development with implications that we are just beginning to work through.
Find Jacob’s Engineering Biology every first and third Friday of the month.
First Five
First Five is our curated list of articles, studies, and publications for the month. For our full list of interesting media in health, science, and technology, updated regularly, follow us on Twitter or Instagram.
1/ From Rats to Mice, to Humans?
The (ugly) naked mole rat is well-known for its extraordinary healthy longevity. A driving element of cancer resistance could be high-molecular-mass hyaluronic acid (HMM-HA). Scientists transferred a gene coding for the enzyme that produces HMM-HA from the naked mole rat into mice and reported remarkable effects, reducing inflammation in multiple tissues and improving overall health. Soon a recipe for healthy aging?
2/ The Optimistic Route
The US has committed to reaching net-zero carbon dioxide emissions by 2050. A group of energy technologists published a set of models laying the path to a whopping 91% reduction in commercial and residential building emissions from the 2005 peak. They highlight how government subsidies can help increase the use of commonly used technologies, such as electric heat pumps and smart thermostats, and go a long way in the right direction.
3/ The Tune Stuck in Your Head
Being able to read the mind has been the subject of countless sci-fi novels and with the progress of computation, scientists have tried ways to decode brain signals and translate them back into readable thoughts. By analyzing an intracranial electroencephalography (iEEG) dataset of 29 patients who listened to a Pink Floyd song, scientists were able to reconstruct a full audio recording from brain activity. Their approach and recordings are available on PLoS Biology website.
4/ Female vs. Male Response to Stress
Males and females respond to stress and psychiatric disorders in vastly different ways. The molecular processes underpinning these differences are still unclear but a team from the Weizmann Institute recently identified the specific cell types responsible for this sexual dimorphism and allowed everyone to interrogate the transcriptomics differences through a convenient web app!
5/ The Great Unknown
While the compendium of human knowledge keeps expanding in biology, thousands of understudied proteins encoded by genes in the human genome, whose existence is known but whose functions are mostly not still exist. Scientists assembled an atlas of these proteins as part of the “unknome” and published them in PLoS Biology.
Digitalis Commons
Catalyzing Health.
Digitalis Commons is a non-profit organization that builds frontier-advancing, scalable solutions that have an outsized impact on important problems in health and health care.
Scientists have been singularly focused on a single metric when deciding where to publish their research: the impact factor. This factor is based on how many times a given paper is cited by other papers. A new study from MIT and other institutions suggests that this measure does not accurately capture medical papers’ impact on patients’ health outcomes, particularly those in low- or middle-income countries. They are proposing a new ‘diversity factor’ and a new platform through which the scientific community can learn about how scientific publications affect the lives of people around the world.
To subscribe to Engineering Biology by Jacob Oppenheim, and receive newly published articles via email, please enter your email address below.